On the Success of the Hadal Snailfishes
The snailfishes, family Liparidae (Scorpaeniformes), have found notable success in the hadal zone from ~6,000-8,200 m. These are the dominant fish group in at least five trenches worldwide. The hadal fish community is distinct from the surrounding abyss where solitary, scavenging fishes such as rattails (Macrouridae), cutthroat eels (Synaphobranchidae), eelpouts (Zoarcidae), and cusk eels (Ophidiidae) are most common.
Little is known about the biology of these deepest-living fishes, nor the factors that drive their success at hadal depths. Using recent collections from the Mariana Trench, Kermadec Trench, and neighboring abyssal plains, some of our research investigates the role of trophic ecology, pressure adaptation, and life history in structuring fish communities at the abyssal-hadal boundary.
Pressure Adaptation
Hadal fishes have developed many adaptations to cope with high hydrostatic pressure. Some metabolic enzymes in hadal snailfishes from the Kermadec and Mariana trenches are adapted to function best at in situ pressures. This has implications for studies of trends of metabolic capacity with depth. Some enzymes may require additional stabilization to function under high pressure, such as with the osmolyte TMAO. Read more here.
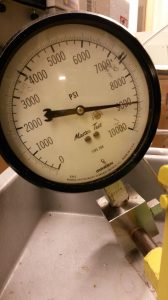
Taxonomy
In 2014, our international team of researchers discovered two new species of hadal snailfishes in the Mariana Trench. We described this new species using morphological and genetic data and gave it an official scientific name, Pseuodoliparis swirei Gerringer & Linley 2017. Check out the full taxonomic description of the Mariana snailfish here.
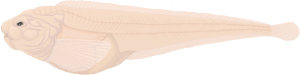
Feeding Ecology
Stomach content and amino acid isotope analyses suggest that suction-feeding predatory fishes like hadal liparids may find an advantage to descending into the trench – where amphipods are abundant. More generalist feeders and scavengers relying on carrion, such as macrourids, might not benefit from this nutritional advantage at hadal depths. Read more here.
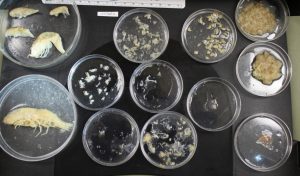
Gelatinous Tissues in Deep-Sea Fishes
Deep-sea fishes have many adaptations to life in their deep-dark world. Some deep-sea fishes are gooey, having a gelatinous layer of tissue below the skin or around the spine. In a study published at Royal Society Open Science, we investigated the function of these gelatinous tissues. Why be made of goo? Read the full paper here. This one involves a robot snailfish!
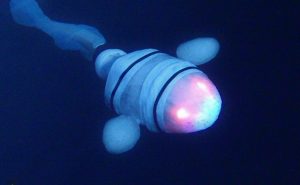
Life History
Otoliths, small calcium carbonate bones in the inner ear of fishes, can give us insight into the life and times of an individual fish. As the fish grows, new opaque rings are deposited in these bones. When we cut the bone, we can see rings like the rings of a tree, allowing us to estimate the age of the fish. Otoliths also retain chemical signatures from the seawater environment. By measuring the composition of the otolith from the inner core to the outside rings, we can look back through the fish’s life. We analyzed otoliths from abyssal and hadal fishes to estimate ages and reconstruct habitat temperatures. Check out the full study here.
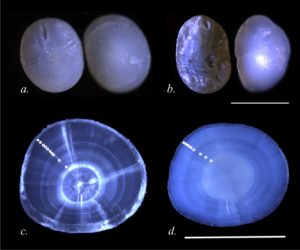
The Pace of Life in Deep-sea Fishes
Jessica Palmeri, Brett Woodworth, Lydia Fregosi, Patrick Flannery, Cassandra Donatelli
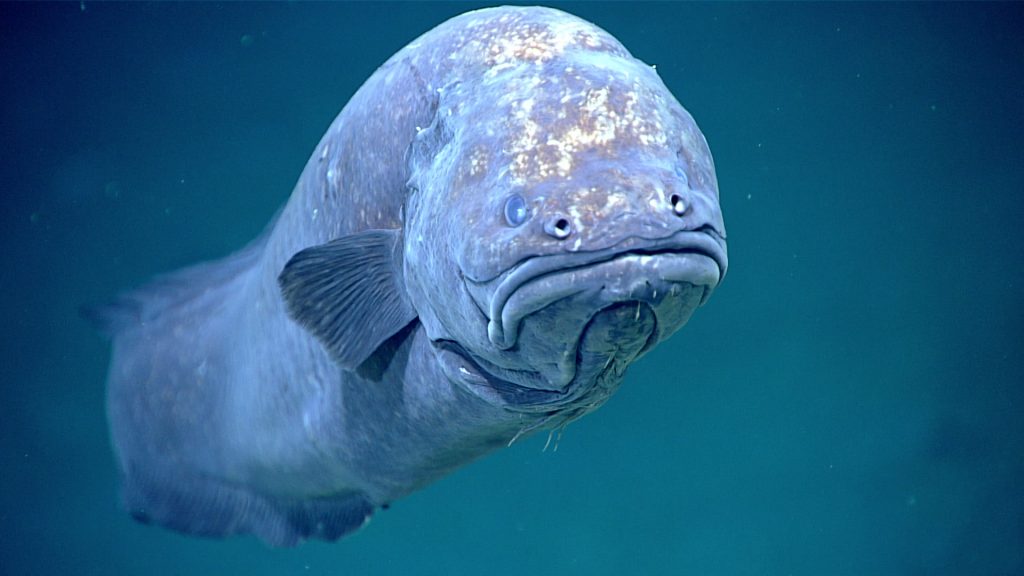
Metabolic rate, the rate at which chemical reactions occur, drives nearly all life processes including growth, reproduction, and development. Within the deep-sea, low temperature and high pressures are thought to impact metabolic rate by slowing the kinetic movement of the particles. Further, when light levels are low, predators cannot see their prey, therefore a fast escape is not necessary. Metabolic rates therefore are expected to decrease as depth increases. However, metabolic rate is difficult to determine in the deep sea. Direct respirometry measurements are challenging in the deep sea so metabolic enzymes are used as proxies for metabolic rate. Enzymes function differently when organisms are taken out of their natural environment, making these proxies an imperfect tool. Therefore, to investigate fish activity with increasing depth, we analyze swimming kinematics in fishes across a large depth gradient (>5000 m) using open source videos from NOAA’s Okeanos Explorer. Through video analysis, we measure head and body lengths, tail beat duration and amplitude, and distance traveled in four major fish families. Metabolism determines the demands that organisms place on their environment and the demands that constrain their own physiology. Understanding the metabolism of deep sea fishes provides insight into energy flow in the deep sea.
The Impact of Seafloor Variance on Biodiversity of Deep-sea Coral and Sponge Habitats
Yumna Ismail, Franchesca Gonzales, Arline Camilo Hernandez, Kayla Cannon
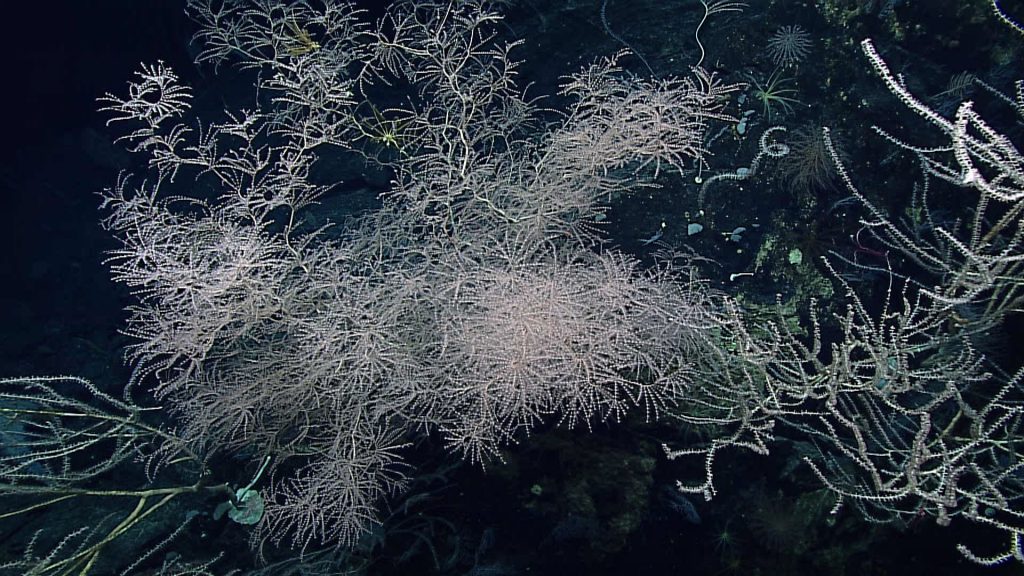
Rugosity – the rough surfaces of the sea floor – generated by coral reefs and rock formations creates a diverse and complex ecosystem that is integral for the survival of shallow-water communities. Little is known about how that translates to the deep sea, which possesses coral ecosystems that are hotspots of biodiversity. We analyzed taxa, sediment type, and rugosity in video segments from NOAA’s Okeanos Explorer’s remotely operated vehicle, taken from the coast of Jarvis Island in the Pacific Remote Islands Marine National Monument. We observed at least 70 unique organism types. According to predictions from our species accumulation curve, there were approximately 106 species observed in the deep-sea off Jarvis Island. We found no significant difference in biodiversity between each rugosity level. Benthic and sessile organisms had a similar number of observations, while pelagic and mobile organisms had more variance. We narrowed our focus to sponges to investigate rugosity effects on sessile fauna. There may be additional factors affecting deep-sea biodiversity, including temperature, presence of predators, food availability, and interspecies relationships. Understanding how small changes in seafloor topography impact ecosystem diversity in deep-sea coral ecosystems could inform management of these vulnerable habitats.
Feeding Modes of Deep-Sea Fishes Based on Jaw Morphologies
Ryan Bohen, Taylor Oleyourryk, Chryssanthi Tzetzis
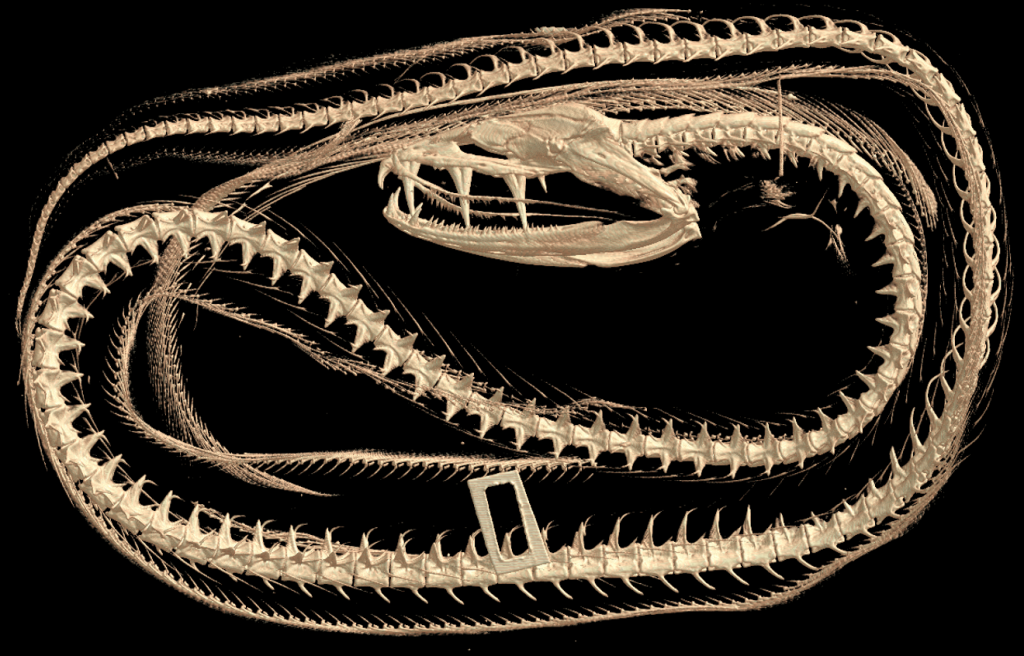
The deep sea is one of the last true frontiers, with its depths remaining relatively unexplored. Deep-sea inhabitants survive high pressures, limited food availability, lack of sunlight, and cold temperatures. These demanding conditions have led to a wide array of adaptations and niches which allows for different ecological roles of fishes. Investigating these deep-sea interactions allows us to better understand the ecosystem as a whole. Our research is investigating feeding biomechanics in deep-sea fishes across three families of fishes, the rattails (Macrouridae), snailfishes (Liparidae), and cusk eels (Ophidiidae). We compare jaw morphologies and mechanics between families to gain insights into feeding mode, including ram feeding (head-on predation), suction feeding, and manipulation. High mechanical advantages in the jaws indicate greater force transmission, characteristic of fishes that manipulate prey, while low mechanical advantage indicates greater velocity transfer, common to suction feeders. We compare tooth shape between the main set of jaws and the pharyngeal set of jaws which aid in prey processing. This research adds new insight into how deep-sea fishes interact in their environment and enhances our understanding of life in the deep oceans, some of the least explored habitats on our planet.